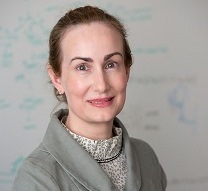
Mollie K. Meffert
725 N. Wolfe Street
413 Physiology Building
Baltimore MD 21205
The goal of the Meffert lab is to gain a mechanistic understanding of how selective gene programs are recruited and maintained to modify the nervous system during development, experience-dependent plasticity, and in injury or disease. Rather than focusing on single genes, we investigate the upstream processes that allow stimuli to dynamically coordinate regulation of the many genes required to mediate changes in growth and excitation. We test molecular mechanisms for spatial control of gene programs at the cellular and subcellular level, with a focus on the roles played by non-coding RNA and RNA-binding proteins. Understanding how genes can be co-regulated post-transcriptionally to produce concerted programs is a fundamental biological question that is also attractive to our laboratory because of its particular relevance for compartmentalized responses in the nervous system, such as forms of synaptic plasticity.
We are highly collaborative and employ multi-disciplinary approaches of molecular diagnostics, biochemistry, high resolution cellular imaging, mouse genetics and behavior, and data science. Discovery-based sequencing strategies have been developed to reveal in vivo small RNA targets through the production of small RNA: target chimeric molecules. The Meffert lab leverages an understanding of basic gene control mechanisms to illuminate potential therapeutic targets in both nerve injury and the development of cognitive disabilities, including Autism Spectrum Disorders such as Fragile X Syndrome, in which disruption of the growth and function of synaptic connections is a central component. Ongoing studies use mouse models and human samples to investigate how translation, miRNA biogenesis and miRNA:target interactions, may be pathologically regulated to produce the aberrant protein synthesis and phenotypes associated with autism.
Mills, W.T. IV, Eadara, S., Jaffe A.E., and Meffert, M.K. (2022) SCRAP: a bioinformatic pipeline for the analysis of small chimeric RNA-seq data rna.079240.122. doi: 10.1261/rna.079240.122.
Mills, W.T. IV, Noor N. Nassar, N.N., Ravindra, D., Li, X, and Meffert, M.K. (2020). Multi-level regulatory interactions between NF-kB and the pluripotency factor Lin28. Cells (special issue), 9 (12);2710. PMID:33348917.
Li, X., Eadara,S., Jeon, S., Liu, Y., Muwanga, G., Qu, L., Caterina, M.J.,and Meffert, M.K. (2021). Combined single-molecule fluorescence in-situ hybridization and immunohistochemistry analysis in intact murine dorsal root ganglia and sciatic nerve. STAR Protocols, DOI: 10.1016/j.xpro.2021.100555
Oldach,L.M., Gorshkov, K., Mills, W.T., Zhang J.*, and Meffert M.K*, (2018), A biosensor for MAPK-dependent Lin28 signaling. Molecular Biology of the Cell, 29(10), 1157-1167.
Dresselhaus, E.C., Boersma, M.C., and Meffert, M.K, (2018), Targeting of NF-kB to dendritic spines is required for synaptic signaling and spine development. J.Neurosci., 8(17); 4093-4103.
Amen, A.M., Ruiz, C.R., Shi J., Subramanian, M., Pham, D.L., and Meffert,M.K. (2017) A rapid induction mechanism for Lin28a in trophic responses. Molecular Cell, 65 (3); 490 – 503.
Subramanian, M., Timmerman, C.K., Schwartz,J.L., Pham,D.L., and Meffert, M.K. (2015), Characterizing Autism Spectrum Disorders by Key Biochemical Pathways. Frontiers in Neuroscience, 9: 313.
Amen,A.M., Pham D.L., and Meffert M.K. (2016) Posttranscriptional regulation by Brain-Derived Neurotrophic Factor in the nervous system. In KMJ Menon and A.Aron Goldstrohm (Ed) Post-transcriptional regulation of endocrine function. Springer Press, p315-337.
Mihalas A.B. and Meffert, M.K. (2015) IKK Kinase Assay for Assessment of Canonical NF-kB Activation in Neurons. Methods in Molecular Biology. v.1280, 61-74.
Ruiz, C.R., Shi, J.,and Meffert, M.K. (2014). Transcript Specificity in BDNF-regulated Protein Synthesis. Neuropharmacol. (Special Issue: BDNF regulation of synaptic structure, function, and plasticity),76; 657-63.
Mihalas, A.B., Araki Y., Huganir, R.L., and Meffert, M.K.(2013), Opposing action of NF-kB and Polo-like kinases determines a homeostatic endpoint for excitatory synaptic adaptation. J.Neurosci.,16; 16490-501.
Huang, Y.A.*, Ruiz, C.R.*, Eyler C.H.*, Lin K., and Meffert, M.K. (2012), Dual regulation of miRNA biogenesis generates target specificity in neurotrophin-induced protein synthesis. Cell, 148(5); 933-946.
Boersma, M.C.*, Dresselhaus, E.C.*, De Biase, L.M., Mihalas, A.B., Bergles, D.E., and Meffert, M.K. (2011), A requirement for NF-kB in developmental and plasticity-associated synaptogenesis. J.Neurosci., 31; 5414-5425.
Shrum, C.K., Defrancisco, D., and Meffert, M.K. (2009) Stimulated nuclear translocation of NF-kB and shuttling differentially depend on dynein and the dynactin complex. PNAS, 106; 2647-2652.
Boersma, M.C., and Meffert, M.K. (2008) Novel roles for the NF-kB signaling pathway in regulating neuronal function. Science Signaling 1, pe7.
Mattson, M.P. and Meffert, M.K. (2006). Roles for NF-kB in nerve cell survival, plasticity, and disease. Cell Death and Differentiation 13, 852-60.
Meffert, M.K. and Baltimore, D. (2005). Physiological functions for brain NF-kB. Trends in Neurosciences 28, 37-43.
Meffert, M.K., Chang, J.M., Wiltgen, B.J., Fanselow, M.S., Baltimore, D. (2003). NF-kB functions in synaptic signaling and behavior. Nature Neuroscience 6, 1072 – 1078.
Meffert, M.K., Calakos, N.C., Scheller, R.H., Schulman H. (1996). Nitric oxide modulates synaptic vesicle docking / fusion reactions. Neuron 16, 1229-1236.
Meffert, M.K. Premack, B.A., and Schulman H. (1994). Nitric oxide stimulates calcium-independent synaptic vesicle release. Neuron 12, 1235-1244.
Meffert, M.K.*, Haley J.E.*, Schuman, E.M., Schulman, H., and Madison, D.V. (1994). Inhibition of hippocampal heme oxygenase, nitric oxide synthase and long-term potentiation by metalloporphyrins. Neuron 13, 1225-1233.